Researchers at North Carolina State University have developed a computational model that helps users understand how changes in the nanostructure of materials affect their conductivity – with the goal of informing the development of new energy storage devices for a wide range of electronics.
Specifically, the researchers were focused on the materials used to make capacitors – which are energy storage devices used in everything from smartphones to satellites.
“You probably use thousands of capacitors in your day-to-day life, whether you know it or not,” says Doug Irving, corresponding author of a paper on the work and an associate professor of materials science and engineering at NC State.
The material that a capacitor is made of affects its performance. So Irving and his collaborators set about developing a model to understand how structural characteristics in a material affect the material’s conductivity.
“One of the things that we’re pleased with is that this model looks at multiple spatial scales simultaneously – capturing everything that is happening from the device-level scale to the nanoscale,” Irving says.
“For example, our model looks at things like defects and grain boundaries,” Irving says. “Defects are things like missing atoms in a material’s structure, or where the ‘wrong’ atoms are found in the structure. Grain boundaries are where different crystalline structures run into each other. Well, our model looks at how things like defects and grain boundaries affect the presence and movement of electrons through a material.
“Because different ways of processing a material can control the presence and distribution of things like defects and grain boundaries, the model gives us insights that can be used to engineer materials to meet the demands of specific applications. In other words, we’re optimistic that the model can help us keep the cost of future capacitors low, while ensuring that they’ll work well and last a long time.”
The paper, “Influence of space charge on the conductivity of nanocrystalline SrTiO3,” is published in the Journal of Applied Physics. First author of the paper is Yifeng Wu, a Ph.D. student at NC State. The paper was co-authored by Preston Bowes and Jonathon Baker, who are both postdoctoral researchers at NC State. The work was done with support from the Air Force Office of Scientific Research, under grants FA9550-14-1-0264 and FA9550-17-1-0318; and support from a Defense Department National Defense Science and Engineering Graduate fellowship.
Abstract
“Influence of space charge on the conductivity of nanocrystalline SrTiO3”
Authors: Yifeng Wu, Preston C. Bowes, Jonathon N. Baker and Douglas L. Irving, North Carolina State University
Published: July 1, Journal of Applied Physics
DOI: 10.1063/5.0008020
A grand canonical multiscale space-charge model has been developed to study and predict the electrical properties of polycrystalline perovskites with complex defect chemistries. This model combines accurate data from hybrid exchange-correlation functional density functional theory calculations (defect formation energies, resultant grand canonical calculations of defect concentrations, and ionization states) with finite-element simulation of the electric field and its coupling to defect redistribution and reionization throughout the grain. This model was used to simulate the evolution of the oxygen partial pressure-dependent conductivity of polycrystalline acceptor-doped strontium titanate as grain size decreases, and the results were compared to previous experiments. These results demonstrate that as the grain size is reduced from microscale to nanoscale, the experimentally observed disappearance of ionic conductivity and forward shift of the oxygen partial pressure of the n-p crossover are successfully reproduced and explained by the model. Mechanistically, the changes to conductivity stem from the charge transfer from the grain boundary core into the grain interior, forming a space charge layer near the grain boundary core that perturbs the local defect chemistry. The impact of grain size on the electrical conductivity and the underlying defect chemistry across the grain are discussed. In addition to the findings herein, the model itself enables exploration of the electrical response of polycrystalline semiconductor systems with complex defect chemistries, which is critical to the design of future electronic components.
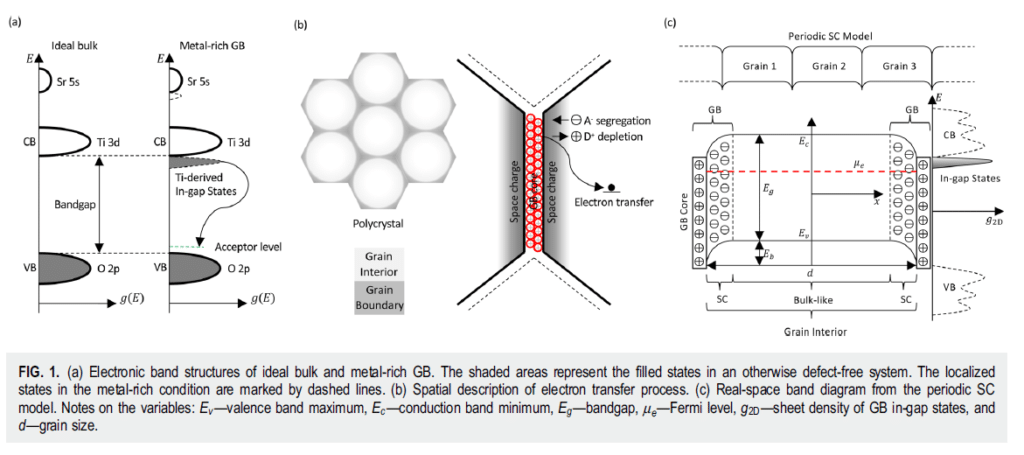