Ferroelectric dielectric materials are getting a serious re-examination related to negative capacitance phenomenon, as semiconductor chipmakers look for new options to maintain drive current.
Ferroelectric materials that are commonly used for ceramic class II dielectric capacitors and piezo devices were one of the highlights of the recent IEEE Electron Device Meeting. They are interesting because a built-in electronic dipole creates a remnant polarization, either P+ or P-.
Applying a strong electric field — the coercive field, Ec — switches the polarization direction, and the new state persists after the field is removed. Ferroelectric memories rely on this effect for non-volatile data storage.
Ferroelectric transistors are a bit more complicated.
They assemble the gate capacitor from a ferroelectric layer (FE) placed in series with a conventional dielectric (DE). Switching the polarization of the FE decreases its charge, leading to a corresponding increase in charge at the DE near the gate. Relative to a conventional MOSFET, this so-called “negative capacitance” effect causes current to increase more rapidly relative to gate voltage, reducing the transistor’s subthreshold swing.
The subthreshold swing (SS), a measure of the steepness of a transistor’s switch from ON to OFF, is the change in voltage needed for a one decade increase in current. As transistors shrink, maintaining an adequate ratio between Ion and Ioff becomes more difficult. A sharper on/off transition is one path to reduced leakage current. Unfortunately, SS in conventional devices is constrained by the Boltzmann limit of about 60mV/decade.
FeFETs have been proposed as a potential solution to this problem. The literature describes these devices as both NCFETs and FeFETs, in part depending on the author’s interpretation of the device physics. (This report uses FeFETs exclusively.) Relative to more radical device architectures, like tunneling FETs, ferroelectric transistors closely resemble conventional MOSFETs. A 2011 demonstration of ferroelectric behavior in hafnium-zirconium oxide (HZO) capacitors showed that ferroelectric materials could be compatible with existing processes. [1]
What is Negative Capacitance ?
Since the negative capacitance effect was first proposed [2], though, researchers have been debating its exact nature. Is it simply a transient switching effect, or evidence of a potentially stable third polarization state?
The argument that negative capacitance is a potentially stable state rests on Landau’s analysis [3] of behavior near a phase transition, as illustrated in Figure 2. In between the stable P+ and P- states, this argument holds, there is a “neutral” configuration that can be maintained by interactions with a conventional dielectric. Controlling the negative capacitance effect in transistors requires accurate matching between the FE and DE layers.
The problem with this analysis, according to Jan Van Houdt, imec’s program director for ferroelectrics, is that the transition between the P+ and P- states corresponds to physical movement of ions in the ferroelectric unit cell. A chemical bond breaks and re-forms; there is no stable state intermediate between the two. Moreover, figure 2 is derived from the Landau-Devonshire model for steady state behavior in equilibrium conditions. Using an equilibrium model to describe switching behavior is inherently problematic.
Instead, discussions of switching dynamics need to consider the forces acting on the material. In the absence of an external field, each ferroelectric unit cell is an electronic dipole surrounded by other dipoles.[4] In a single crystal the lowest energy state will be one in which all of the dipoles are aligned in the same direction. In HZO deposited on HfO2 or silicon, a more likely result is a polycrystalline material, with grain boundaries and somewhat random crystal orientations. The P+ direction for one grain may not align with the P+ direction of its neighbors. Depending on the deposition conditions, there may even be crystallites that are not ferroelectric at all. [5] The net polarization of the material is the sum of the P+ and P- domains.
When an electric field is applied, the dipoles begin to align themselves with it. Switching of individual dipoles is very fast — one of the fastest electronic switching mechanisms known — but not all domains in a multi-crystalline, randomly oriented film will switch at once. Shifting the net polarization from P+ to P- or vice versa requires finite time.
Read more about negative capacitance in post here: Negative Capacitance in Action
Optimizing subthreshold swing
While the switching is taking place, the change in polarization leads to a change in the net capacitance of the material. Maintaining a constant voltage requires an influx of charge from an external source: current flows. Huimin Wang and colleagues at Peking University explained that negative capacitance behavior thus occurs when the rate of change of the polarization is greater than the rate of change of the capacitance. [6] They observed the effect in standalone FE capacitors, indicating that the presence of a DE layer is not fundamental to the effect. When a ferroelectric is in series with a conventional dielectric, though, the interaction between the two will define the overall electrostatics of the device.
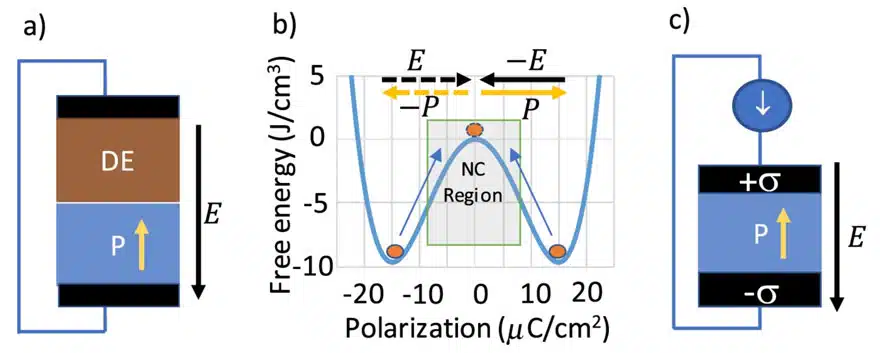
Even if there is no persistent negative capacitance state, it is possible to have negative differential capacitance. Depending on the sweep rate of the gate voltage, the net capacitance may increase sharply, then fall as the gate voltage “catches up” and Vth is reached.
Negative capacitance (NC) can be stabilized by putting a non-ferroelectric capacitor in series with the FE. When the voltage across the stack is zero as shown in Fig. 2(a), the negative sign indicates that the electric field acts against the polarization. Such field is called the depolarization field. Depolarization field pushes the polarization state from the minima towards the top of the Landau free energy landscape. Consequently it acts as a stabilization mechanism that keeps the FE in the NC region as shown in Fig. 2(b). Note that this is not the only way to establish a depolarization field. The electric field inside a FE arises from the surface charge density σσ supplied from an external source as shown in Fig. 2(c).
Whether ferroelectric behavior is relevant for commercial devices therefore remains an open question.
For memory, (to be discussed in Part II of this series), the answer seems to be unequivocally, yes. Rapid, persistent switching places FeRAM in an important niche between flash memory and DRAM. For transistors, though, the answer is less clear. While using negative capacitance to enhance MOSFET performance may not be possible, some 2D semiconductor materials also have ferroelectric characteristics. Part III in this series will consider what role ferroelectrics might play in advanced channel devices.
References
- 1. J. Müller, et. al., “Ferroelectric Zr0.5Hf0.5O2 thin films for nonvolatile memory applications”, Appl. Phys. Lett. 99, 112901 (2011) https://doi.org/10.1063/1.3636417
- 2. Sayeef Salahuddin and Supriyo Datta, “Use of Negative Capacitance to Provide Voltage Amplification for Low Power Nanoscale Devices”, Nano Letters 2008 8 (2), 405-410 DOI: 10.1021/nl071804g
- 3. P. Chandra and P.B. Littlewood, “A Landau Primer for Ferroelectrics.” https://doi.org/10.48550/arXiv.cond-mat/0609347
- 4. Alam, M.N.K., Roussel, P., Heyns, M. et al. “Positive non-linear capacitance: the origin of the steep subthreshold-slope in ferroelectric FETs.” Sci Rep 9, 14957 (2019). https://doi.org/10.1038/s41598-019-51237-2
- 5. M. Dragoman, et. al., “The Rise of Ferroelectricity at Nanoscale: Nanoelectronics is rediscovering the ferroelectricity,” in IEEE Nanotechnology Magazine, vol. 15, no. 5, pp. 8-19, Oct. 2021, doi: 10.1109/MNANO.2021.3098217.
- 6. H. Wang et al., “New Insights into the Physical Origin of Negative Capacitance and Hysteresis in NCFETs,” 2018 IEEE International Electron Devices Meeting (IEDM), 2018, pp. 31.1.1-31.1.4, doi: 10.1109/IEDM.2018.8614504.